Before continuing this article, get our free ABG Quiz
Disorders of acid–base balance can lead to severe complications in many disease states, and occasionally the abnormality may be so severe as to become a life-threatening risk factor. The process of analysis and monitoring of arterial blood gas (ABG) is an essential part of diagnosing and managing the oxygenation status and acid–base balance of the high-risk patients, as well as in the care of critically ill patients in the Intensive Care Unit. Since both areas manifest sudden and life-threatening changes in all the systems concerned, a thorough understanding of acid–base balance is mandatory for any physician, and the anesthesiologist is no exception. However, the understanding of ABGs and their interpretation can sometimes be very confusing and also an arduous task. Many methods do exist in literature to guide the interpretation of the ABGs. The discussion in this article does not include all those methods, such as analysis of base excess or Stewart’s strong ion difference, but a logical and systematic approach is presented to enable us to make a much easier interpretation through them. The proper application of the concepts of acid–base balance will help the healthcare provider not only to follow the progress of a patient, but also to evaluate the effectiveness of care being provided.
Introduction
Arterial blood gas (ABG) analysis is an essential part of diagnosing and managing a patient’s oxygenation status and acid–base balance. The usefulness of this diagnostic tool is dependent on being able to correctly interpret the results. Disorders of acid–base balance can create complications in many disease states, and occasionally the abnormality may be so severe so as to become a life-threatening risk factor. A thorough understanding of acid–base balance is mandatory for any physician, and intensivist, and the anesthesiologist is no exception.
The three widely used approaches to acid–base physiology are the HCO3– (in the context of pCO2), standard base excess (SBE), and strong ion difference (SID). It has been more than 20 years since the Stewart’s concept of SID was introduced, which is defined as the absolute difference between completely dissociated anions and cations. According to the principle of electrical neutrality, this difference is balanced by the weak acids and CO2. The SID is defined in terms of weak acids and CO2 subsequently has been re-designated as effective SID (SIDe) which is identical to “buffer base.” Similarly, Stewart’s original term for total weak acid concentration (ATOT) is now defined as the dissociated (A–) plus undissociated (AH) weak acid forms. This is familiarly known as anion gap (AG), when normal concentration is actually caused by A–. Thus all the three methods yield virtually identical results when they are used to quantify acid–base status of a given blood sample.[1]
Why is it Necessary to Order an ABG Analysis?
The utilization of an ABG analysis becomes necessary in view of the following advantages:
- Aids in establishing diagnosis.
- Guides treatment plan.
- Aids in ventilator management.
- Improvement in acid/base management; allows for optimal function of medications.
- Acid/base status may alter electrolyte levels critical to a patient’s status.
Accurate results for an ABG depend on the proper manner of collecting, handling, and analyzing the specimen. Clinically important errors may occur at any of the above steps, but ABG measurements are particularly vulnerable to preanalytic errors. The most common problems that are encountered include nonarterial samples, air bubbles in the sample, inadequate or excessive anticoagulant in the sample, and delayed analysis of a noncooled sample.
Obtain a relevant clinical history
While making an interpretation of anABG, never comment on the ABG without obtaining a relevant clinical history of the patient, which gives a clue to the etiology of the given acid–base disorder. For example, a patient with a history of hypotension, renal failure, uncontrolled diabetic status, of treatment with drugs such as metformin is likely to have metabolic acidosis; a patient, with a history of diuretic use, bicarbonate administration, high-nasogastric aspirate, and vomiting, is likely to have metabolic alkalosis. Respiratory acidosis would occur in COPD, muscular weakness, postoperative cases, and opioid overdose, and respiratory alkalosis is likely to occur in sepsis, hepatic coma, and pregnancy.
Look at the oxygenation status of the patient
The oxygenation status of the patient is judged by the paO2; however, never comment on the oxygenation status without knowing the corresponding FiO2. Calculate the expected paO2 (generally five times the FiO2).[6]
Based on the expected paO2 classify as mild, moderate, and severe hypoxia.
- Ventilatory status: Look at paCO2.
- Acid–base status: Identify the primary disorder by looking at the pH
pH > 7.40–Alkalemia: – 7.40-Acidemia
Then look at paCO2 which is a respiratory acid, whether it is increased, i.e., >40 (acidosis) or decreased <40 (alkalosis) and if this explains the change of pH, then it is respiratory disorder; otherwise, see the trend of change of HCO3–(whether increased in alkalosis or decreased in acidosis)–if it explains the change of pH, then it is a metabolic disorder.
In a normal ABG
- pH and paCO2 move in opposite directions.
- HCO3–and paCO2 move in same direction.
-
When the pH and paCO2 change in the same direction (which normally should not), the primary problem is metabolic; when pH and paCO2 move in opposite directions and paCO2 is normal, then the primary problem is respiratory.
-
Mixed Disorder–if HCO3– and paCO2 change in opposite direction (which they normally should not), then it is a mixed disorder: pH may be normal with abnormal paCO2 or abnormal pH and normal paCO2).[7]
If the trend of change in paCO2 and HCO3– is the same, check the percent difference. The one, with greater % difference, between the two is the one that is the dominant disorder.
e.g.: pH = 7.25 HCO3–=16 paCO2=60
Here, the pH is acidotic and both paCO2 and HCO3– explain its acidosis: so look at the % difference
HCO3–% difference = (24 – 16)/24 = 0.33
paCO2% difference = (60 – 40)/40 = 0.5
Therefore, respiratory acidosis as the dominant disorder.
Respiratory disorders
After the primary disorder is established as respiratory, then the following points will help us to approach further with regard to the respiratory disorder).[8]
- Ratio of rate of change in H+to change in paCO2
- Alveolar arterial oxygen gradient
- Compensation
Ratio of rate of change in H+to change in paCO2
The above ratio of rate of change in H+to change in paCO2 helps in guiding us to conclude whether the respiratory disorder is acute, chronic, or acute on chronic. As we have seen, the hydrogen can be calculated from Table 1 and the change in H+ is calculated by subtracting the normal H+ from the calculated H+ ion.[9]
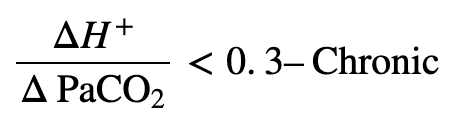
>0.8–acute
0.3–0.8–acute on chronic
Alveolar Arterial Oxygen Gradient
It is calculated as follows:
where PAO2, alveolar partial pressure of oxygen; PiO2, partial pressure of inspired oxygen; FiO2, fraction of inspired oxygen; PB, barometric pressure (760 mmHg at sea level); PH2O, water vapor pressure (47 mm Hg), PaCO2, partial pressure of carbon dioxide in blood; R, respiratory quotient assumed to be 0.8.

Hypoxemic respiratory failure can be associated with normal (10–15 mmHg) or increasedalveolar arterial oxygen gradient. Figure 2 shows the alogrithim for approach in a patient with hypoxemic respiratory failure. If this gradient is <20, then it indicates an extrapulmonary cause of respiratory failure.

-
Central nervous system–Respiratory center depression due to causes such as drug overdose, primary alveolar hypoventilation, and myxedema.
-
Peripheral nervous system–Spinal cord diseases, Guillain-Barré syndrome, Amyotrophic lateral sclerosis.
-
Respiratory muscles–Hypophosphatemia, muscle fatigue, myasthenia gravis, and polymyositis.
-
Chest wall diseases–Ankylosing spondylitis, flail chest, thoracoplasty.
-
Pleural diseases–Restrictive pleuritis
-
Upper air way obstruction–Tracheal Stenosis, vocal cord tumor
Compensation
Rules of compensation
-
The compensatory response depends upon the proper functioning of the organ system involved in the response (lungs or kidneys) and on the severity of acid–base disturbance. For example, the likelihood of complete compensation in chronic respiratory acidosis is <15% when paCO2 exceeds 60 mmHg.
-
Acute compensation occurs within 6–24 h and chronic within 1–4 days. Respiratory compensation occurs faster than metabolic compensation.
-
In clinical practice, it is rare to see complete compensation. The maximum compensatory response in most cases is associated with only 50–75% return of pH to normal. However, in chronic respiratory alkalosis, the pH may actually completely return to normalcy in some cases.
Respiratory acidosis
Acute: [HCO3–] increase by 1 mEq/L for every 10 mmHg increase in paCO2 above 40.
Chronic: [HCO3–] increase by 3.5 mEq/L for every 10 mmHg increase in paCO2 above 40.
Respiratory alkalosis
Acute: [HCO3–] decrease by 2 mEq/L for every 10 mmHg decrease in paCO2 below 40.
Chronic: [HCO3–] decrease by 5 mEq/L for every 10 mmHg decrease in paCO2 below 40.
Metabolic disorders
In patients with metabolic acidosis, an excess of acid or loss of base is present. This causes the HCO3–:H2CO3ratio and pH to fall while no change occurs in pCO2–uncompensated metabolic acidosis.
As a result of compensatory mechanisms, the lungs in the form of CO2 excrete H2CO3 and the kidneys retain HCO3–. pCO2 falls and HCO3–: H2CO3 ratio and pH rise toward normal even though concentrations of HCO3–and H2CO3 are less than normal. This is called compensated metabolic acidosis and the expected paCO2 is calculated as paCO2 = [1.5 × HCO3+ 8] ± 2.
Anion gap
For more than 40 years, the AG theory has been used by clinicians to exploit the concept of electroneutrality and has evolved as a major tool for evaluating the acid–base disorder. Anion gap is the difference between the charges of plasma anions and cations, calculated from the difference between the routinely measured concentration of the serum cations (Na+ and K+) and anions (Cl– and HCO3–). Because electroneutrality must be maintained, the difference reflects the unmeasured ions. Normally, this difference or the gap is filled by the weak acids (A–) principally albumin, and to a lesser extent phosphates, sulfates, and lactates.
When the AG is greater than that produced by the albumin and phosphate, other anions (e.g., lactates and ketones) must be present in higher than normal concentration.
Anion gap = (Na+ + K+) – [Cl– + HCO3–]
Because of its low and narrow extracellular concentration, K+ is often omitted from the calculation The normal value ranges from 12 ± 4 when K+ is considered, and 8 ± 4 when K+ is omitted. Figure 3 shows the alogrithm for the approach to patients with normal AG acidosis.

The primary problem with AG is its reliance on the use of the normal range produced by the albumin and to a lesser extent phosphate, the level of which may be grossly abnormal in critically ill patients. Because these anions are not strong anions, their charges will be altered by changes in pH.[10,11]
Serum protein and phosphate
Normal AG = 2{albumin(gm/L)} + 0.5 {phosphate (mg/dL)}
Acid–base status
In Acidemic state – Anion gap decreases by 1-3
In Alkalemic state – Anion gap increases by 2-5
Major clinical uses of the anion gap
- For signaling, the presence of a metabolic acidosis and confirm other findings.
- Helping to differentiate between causes of metabolic acidosis: High AG versus normal AG metabolic acidosis. In an inorganic metabolic acidosis (e.g., due to HCl infusion), the infused Cl– replaces HCO3–, and the AG remains normal. In an organic acidosis, the lost bicarbonate is replaced by the acid anion which is not normally measured. This means that the AG is increased.
- Providing assistance in assessing the biochemical severity of the acidosis and follow the response to treatment.
Disorders that are associated with a low or negative serum AG are listed in Table 2.
Table 2
Disorders associated with low serum anion gap
Cause | Comments |
---|---|
Laboratory error | Most frequent cause of low anion gap |
Hypoalbuminemia | Second most common cause of low serum anion gap |
Multiple myeloma | Level of anion gap correlates with serum concentration of paraprotein |
Halide intoxication | Anion gap depends on serum halide concentration |
(bromide, lithium, iodide) | (low anion gap with lithium ≥4 mEq/L) |
Hypercalcemia | more likely in hypercalcemia associated with 10 hyperparathyroidism |
Hypermagnesemia | Theoretical cause but not documented in literature |
Polymyxin B | Anion gap depends on serum level; occurs with preparation with chloride |
Underestimation of serum sodium | Most frequent with hypernatremia or hypertriglyceridemia |
Overestimation of serum chloride | Rare with ion selective electrodes |
Overestimation of serum bicarbonate | Spurious in serum HCO3 if cells not separated from sera |
Table 3 elaborates the species of the unaccounted anions along with their sources of origin and diagnostic adjunts in case of high AG metabolic acidosis.
Table 3
Description of the species of unmeasured anions, source of origin, and diagnostic adjuncts in case of high anion gap metabolic acidosis
Cause | High serum anion gap
|
||
---|---|---|---|
Comments
|
|||
Species | Origin | Diagnostic adjuncts | |
Renal failure | Phosphates, sulphates | Protein metabolism | BUN/creatinine |
Ketocidosis | Ketoacids | Fatty acid metabolism | Serum/urine ketones |
Diabetic | β Hydroxybutyrate | ||
Alcoholic | |||
Starvation | Acetoacetate | ||
Lactic acidosis | Lactate | Lactate levels | |
Exogenous poisoning | Salicylate | Salicylate | Concomitant |
Lactate | Respiratory and metabolic alkalosis | ||
ketoacids |
In the patients with metabolic alkalosis, there is an excess of base or a loss of acid which causes the HCO3–:H2CO3 ratio and pH to rise, but with no change occurring in pCO2, which is called uncompensated metabolic alkalosis. However, the kidney has a large capacity to excrete excess bicarbonate and so, for sustaining the metabolic alkalosis, the elevated HCO3–concentration must be maintained through an abnormal renal retention of HCO3–.
Compensatory respiratory acidosis may be so marked that pCO2 may rise higher than 55 mmHg. Expected paCO2is calculated as paCO2 = [0.7 × HCO3–+ 21] ± 2 or 40 + [0.7 ΔHCO3]. This is called compensated metabolic alkalosis.
Most of the patients with metabolic alkalosis can be treated with chloride ions in the form of NaCl (saline responsive) rather than KCl (which is preferable). When NaCl is given, Cl–ions are supplied, and so the blood volume increases and the secretion of aldosterone in excess decreases. Thus, excessive urinary loss of K+and excessive reabsorption of HCO3– stops. When metabolic alkalosis is due to the effects of excessive aldosterone or other mineralocorticoids, the patient does not respond to NaCl (saline resistant) and requires KCl.
Based on the urinary chloride, metabolic alkalosis is divided into:
Chloride responsive or extracellular volume depletion (urinary chloride < 20)
- Vomiting
- Diuretic
- Post hypercapnic
- Chronic diarrhea
- Chloride resistant (urinary chloride > 20)
- Severe potassium depletion
- Mineralocorticoid excess–Primary hypealdosteronism, Cushing’s Syndrome, Ectopic ACTH
- Secondary hypereldosteronism–Renovascular disease, malignant hypertension, CHF, cirrhosis
Aproach to mixed disorder
Mixed metabolic disturbances (e.g., high AG from diabetic ketoacidosis plus normal AG from diarrhea) can be identified using the relationship between AG and HCO3–, which is called the gap–gap ratio. It is the ratio of change in anion gap (ΔAG) to change in HCO3– (ΔHCO3–).
When hydrogen ions accumulate in blood, the decrease in serum HCO3– is equivalent to the increase in AG and the increase in AG excess/HCO3– deficit ratio is unity, i.e., pure increase in AG metabolic acidosis. When a normal AG acidosis is present, the ratio approaches zero.
When a mixed acidosis is present (high AG + normal AG), the gap–gap ratio indicates the relative contribution of each type to the acidosis. If it is <1, then it suggests that there is a normal AG metabolic acidosis associated with it and if >2 it suggests that there is associated metabolic acidosis.
Rules for rapid clinical interpretation of ABG
When required to make a proper approach towards the evaluation of blood gas and acid–base disturbances in the body, the following scheme is suggested:
-
Look at pH – < 7.40 – Acidosis; > 7.40 – Alkalosis
-
If pH indicates acidosis, then look at paCO2and HCO3–
-
If paCO2is ↑, then it is primary respiratory acidosis
-
To determine whether it is acute or chronic
ΔH+ / ΔpaCO2 <0.3–chronic
>0.8–acute
0.3-0.8–acute on chronic
-
Calculate compensation by the respective methods
Acute: [HCO3–] ↑ by 1 mEq/L for every 10 mmHg ↑ in paCO2 above 40.
Chronic: [HCO3–] ↑ by 3.5 mEq/L for every 10 mmHg ↑ in paCO2 above 4
-
-
If paCO2↓ and HCO3– is also ↓→ primary metabolic acidosis
Calculate expected paCO2as follows:
paCO2 = [1.5 × HCO3+ 8] ± 2 metabolic acidosis only
paCO2 < expected paCO2→ concomitant respiratory alkalosis.
paCO2 > expected paCO2→ concomitant respiratory acidosis
-
If HCO3–is ↓, then AG should be examined.
-
If AG is unchanged → then it is hyperchloremic metabolic acidosis.
-
If AG is ↑ → then it is wide AG acidosis.
-
Check gap-gap ratio
ΔAG/Δ HCO3– = 1, pure increased AG metabolic acidosis
<1 normal anion gap metabolic acidosis
>2 associated metabolic acidosis.
-
If pH indicates alkalosis, then look at HCO3– and paCO2.
-
If paCO2is ↓ → then it is primary respiratory alkalosis.
-
Whether it is acute or chronic (with the same formula as above)
-
Calculate compensation by the respective methods:
Acute: [HCO3–]↓ by 2 mEq/L for every 10 mmHg
↓ in paCO2below 40.
Chronic: [HCO3–] ↓ by 5 mEq/L for every
10mmHg ↓ in paCO2 below 40.
-
-
If paCO2 ↑ and HCO3– also ↑ → then it is primary metabolic alkalosis.
Calculate the expected paCO2
paCO2 = [0.7 × HCO3–+ 21] ± 2 Or 40 + [0.7 ΔHCO3] → metabolic alkalosis only
paCO2 < expected paCO2 → concomitant respiratory alkalosis.
paCO2 > expected paCO2 → concomitant respiratory acidosis
-
Check urinary chloride
if urinary chloride < 20 → chloride responsive or ECV depletion
if urinary chloride > 20→ chloride resistant
-
If pH is normal ABG may be normal or mixed disorder
-
↑paCO2 and ↓HCO3–→ respiratory and metabolic acidosis
-
(b) ↓paCO2 and↑ HCO3–→ respiratory and metabolic alkalosis.
Calculate % difference (ΔHCO3–/HCO3–and ΔpaCO2/paCO2) to see which is dominant disorder.
-
Examples on ABG interpretation:
Example 1:
A 17-year-old patient presents to A&E complaining of a tight feeling in their chest, shortness of breath and some tingling in their fingers and around their mouth. They have no significant past medical history and are not on any regular medication. An ABG is performed on the patient who is not currently receiving any oxygen therapy.
The ABG result shows the following:
- PaO2: 14 (11-13 kPa) || 105 mmHg (82.5 – 97.5 mmHg)
- pH: 7.49 (7.35 – 7.45)
- PaCO2: 3.6 (4.7-6.0 kPa) || 27 mmHg (35.2 – 45 mmHg)
- HCO3-: 24 (22-26 mEq/L)
Answer:
Oxygenation (PaO2)
A PaO2 of 14 on air is at the upper limit of normal, so the patient is not hypoxic.
pH
A pH of 7.49 is higher than normal and therefore the patient is alkalotic. The next step is to figure out whether the respiratory system is contributing the alkalosis (e.g. ↓ CO2).
PaCO2
The CO2 is low, which would be in keeping with an alkalosis, so we now know the respiratory system is definitely contributing to the alkalosis, if not the entire cause of it. The next step is to look at the HCO3– and see if it is also contributing to the alkalosis.
HCO3–
HCO3– is normal, ruling out a mixed respiratory and metabolic alkalosis, leaving us with an isolated respiratory alkalosis.
Compensation
There is no evidence of metabolic compensation of the respiratory alkalosis (which would involve a lowered HCO3-) suggesting that this derangement is relatively acute (as metabolic compensation takes a few days to develop).
Answer
Respiratory alkalosis with no metabolic compensation. The underlying cause of respiratory alkalosis, in this case, is a panic attack, with the hyperventilation, peripheral and peri-oral tingling being classical presenting features.
Example 2:
A 16-year-old female presents to hospital with drowsiness and dehydration. They have no previous past medical history and are on no regular medication. An ABG is performed on room air.
- PaO2: 14 (11-13 kPa) ||105 mmHg (82.5 – 97.5 mmHg)
- pH: 7.33 (7.35 – 7.45)
- PaCO2: 3.0 (4.7-6.0 kPa) || 22.5 mmHg (35.2 – 45 mmHg)
- HCO3-: 17 (22-26 mEq/L)
Answer:
- Oxygenation (PaO2)
- A PaO2 of 14 on air is at the upper limit of normal, so the patient is not hypoxic.
- pH
- A pH of 7.33 is lower than normal and therefore the patient is acidotic. The next step is to figure out whether the respiratory system is contributing the acidosis (i.e. ↑ CO2).
- PaCO2
- The CO2 is low, which rules out the respiratory system as the cause of the acidosis (as we would expect it to be raised if this was the case). So we now know the respiratory system is NOT contributing to the acidosis and this is, therefore, a metabolic acidosis. The next step is to look at the HCO3– to confirm this.
- HCO3–
- HCO3– is low in keeping with a metabolic acidosis.
- Compensation
- We now know that the patient has a metabolic acidosis and therefore we can look back at the CO2 to see if the respiratory system is attempting to compensate for the metabolic derangement. In this case, there is evidence of respiratory compensation as the CO2 has been lowered in an attempt to normalise the pH. An important point to recognise here is that although the derangement in pH seems relatively minor this should not lead to the assumption that the metabolic acidosis is also minor. The severity of the metabolic acidosis is masked by the respiratory system’s attempt at compensating via reduced CO2 levels.
- Answer
- Metabolic acidosis with respiratory compensation. The underlying cause of the metabolic acidosis, in this case, is diabetic ketoacidosis.
Get our free ABG Quiz
Top 5 Amazon books on ABG interpreation:
Summary of ABG interpretation
Youtube video about ABG interpretation
Take our general medicine quizzes: General Medicine MCQs